THE MIDDLE
But what stops a star itself from collapsing? Well
here we are back to pressure. But, we could argue, because stars are always
loosing energy by radiation (that is why we see them) they will therefore
collapse in the way we described earlier, albeit over a much longer time
scale than if they were in free-fall. The fact that they do not is that
they can draw on a source of energy other than gravitational potential
energy. This source is nuclear energy. In the very hot, very dense gas at
the centres of stars, the motions of the nuclei are so energetic that they
have sufficient velocities to break through the electrostatic repulsive
forces which exist between the nuclei (which are all positively charged).
When they are very close, the short range nuclear force comes into play and
the nuclei can fuse together releasing enormous amounts of energy. This
energy replenishes the energy lost from the surface of the star and thus
maintains the internal pressure within the star which opposes its collapse.
In this way the light elements such as hydrogen are converted into heavier
elements and so on. This can occur all the way up to iron which is the
most stable element. This process is called the nucleosynthesis of the
elements and is the main way by which the heavier elements are formed.
Because the elements in your body are heavy, you (or rather the atoms in
your body) must at some stage have been at the centre of a star.
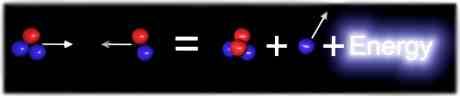
IMAGE - The fusion process
A deuteron (a hydrogen nucleus with a neutron) and a triton (a hydrogen nucleus with two neutrons) fuse together to a form a helium nucleus, a neutron and excess energy. This energy produces the heat and light for the star.
Nuclear fusion only occurs at very high temperatures (of order a million degrees or higher). How does the centre of a star get this hot in the first place? This is by converting the gravitational potential of the large gas cloud into heat (which is kinetic energy). Stars in which nuclear burning takes place in their centres are called Main Sequence stars of which our Sun is one. It is about a million miles across (or about two light seconds). The masses of main sequence stars range from about ten times the mass of the Sun to about a tenth of the mass of the Sun and are called Giants and Dwarfs respectively. The mass of a star, through its gravitational potential energy, determines the central temperature of the star. Stars with hotter centres burn more rapidly and therefore have hotter photospheres (surfaces) as well. Hence the Blue Giants are observed to be very hot and the Red Dwarfs are relatively cool.
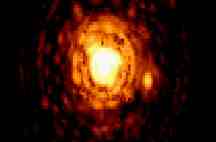
IMAGE - picture of a red dwarf star taken by the Hubble Space Telescope
Some collapsing clouds never contained enough mass (and hence gravitational energy) to ever get hot enough to become stars. The gas became sufficiently dense to become a solid before the central temperatures could reach the million mark and as we know solids do not readily contract and so the collapse process was arrested. These objects are called Brown Dwarfs and are similar to giant planets. It is thought that there may be very many of these in the Universe; they are small, and when they have cooled down they are non-luminous so they are very difficult to see.
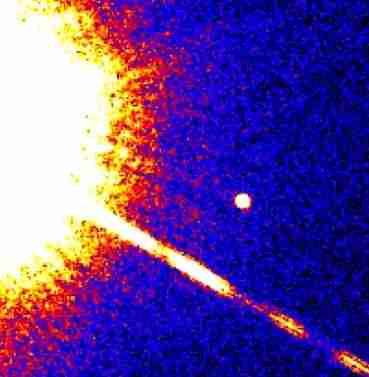
IMAGE - first ever image of a brown dwarf
Since brown dwarfs are not luminous, they are extremely hard to observe. In this image, you can just about see the brown dwarf to the right of the main star.
At some stage the centre of a star will be depleted of its nuclear fuel and the overall collapse of the star can continue. Things are slightly more complicated than this and first the star goes through an expansion phase where it becomes a Red Giant. Our Sun will do this in the next 5 billion years and will expand so much its surface will reach out to the distance of the Earth; indeed the Earth will probably be evaporated by the process. This expansion will be followed eventually by another collapse followed by another expansion. This cycle may repeat itself for a number of times until the expansion phase is not arrested and the Sun manages to blow off a large part of its atmosphere. At this point it becomes a Planetary Nebula.
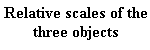
IMAGE - The future of our Sun Our Sun will turn into a red giant in a few billions of years. It will then blow away a large part of its atmosphere and become a white dwarf. The final picture shows a planetary nebula with a white dwarf at its centre.
Having lost its outer envelope, the evolved centre of the Red Giant will be exposed. This is the White Dwarf which is about the size of the Earth (and is indeed solid) and contains a large fraction of the mass of the Sun. It is very hot (about 100,000 degrees) but because it is solid it does not do very much other than cool. At this stage the star is essentially dead.
Without going into any detail, a number of other exciting things can occur in these death throes of stars. They can end up even smaller as Neutron Stars (about 10 miles across) or even Black Holes (which are vanishingly small). These are the so-called compact objects. Neutron stars are stars in which the gravitational forces are balanced by the repulsive, very short range, nuclear forces. But after this there are no more forces in nature left and gravity can finally have its "evil way"; and the Black Hole is the result. Here is an example of how gravity can win out essentially leaving us with nothing.
However, there is an alternative final demise which in fact turns out not to be final at all. So much gravitational energy can be released in the final collapse that the star can simply explode to form a Supernova in which the entire mass of the star is spewed back into interstellar space ultimately forming a Supernova Remnant. This energy released in this massive explosion can also be used to form the very heavy trans-iron elements. Although heavier than iron they are less stable and can eventually yield up some of this added energy through stabilising, nuclear decay processes. An example of this is fission and it is by this process that we get energy from Uranium and Plutonium in our nuclear power stations and which is fed into the national electricity grid.
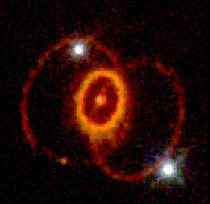
IMAGE - Supernova 1987a
In a way it is ironic that the supernova explosion is the ultimate way to prevent matter from collapsing into itself. It essentially puts us back where we started though with one main difference. In this overall cycle heavier elements have been formed from the lighter elements. The star formation process can now start again. Indeed, the fact that we can see iron in the surface of our own Sun tells us that it cannot have been a first generation star but must have formed out of matter previously processed in another star.
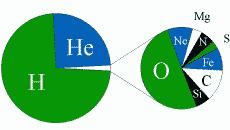
IMAGE - A pie chart representing the composition of the Sun
By examining the composition of the Sun, scientists believe that the material in our Sun has been through at least two supernovae
We have now essentially covered all the mechanisms by which matter is prevented from falling in on itself; pressure (making use of electromagnetic and nuclear forces), orbital motion, and expansion.
|