PHOTONS AND LIGHT

Why is light important?
The interaction between
light and matter is important to all aspects of physics and especially
so to astronomy. Astronomers obtain all but a tiny fraction of their
information about the universe in the form of electromagnetic
radiation and it is only by understanding exactly how it interacts
with the matter in the universe that we can hope to accurately
determine the physical properties of the objects we see. To be able to
do this we need a model which is capable of describing the behaviour
of electromagnetic radiation in an entirely self-consistent fashion
and such a theory has been surprisingly difficult to obtain. It is
usually fairly easy to describe any single particular phenomenon but
it is self-consistency which has been hard to provide. It is this
strange behaviour of light and attempts to understand it that have
been crucial to the development of the world of quantum
mechanics.

How have theories of light evolved?
Historically - until
around 300 years ago, scientists viewed light as a stream of particles
with each individually carrying a small amount of energy. However, as
more and more experiments with light were being performed it became
apparent that this corpuscular model was unable to describe many of
light's properties - diffraction, refraction and so on, and it
eventually became clear that light was better described as a wave
phenomenon. This view of light was strengthened when James Maxwell put
forward a working theory as to the nature of these waves being
oscillations of both the electric and magnetic fields - hence the name
electromagnetic radiation.
However, at the beginning
of this century it became clear that there were other aspects of
light's behaviour, such as the photo-electric effect, that could not
be described by the action of waves and it was necessary to re-invoke
the particle theory of light. Both wave and particle descriptions are
necessary to describe how light interacts with matter and the most
appropriate visualization is dependent on the exact situation
involved, this strange property is known as wave-particle
duality. When we consider waves we envisage light as oscillations in
electric and magnetic fields. When we consider the particle nature we
view light as a stream of individual packets, or quanta, of
energy. Each individual packet is called a PHOTON.

What is the photo-electric effect?
The photo-electric effect is the name given to the phenomenon whereby
metals eject electrons in response to light falling on their
surface. Because of the way the different atoms link together in a metal
some of the electrons are largely free to move around the atomic
lattice. These electrons are not strongly attached to individual atoms
and are what give metals their properties. The electrons can easily be
moved by the application of an electric field and this is what makes
metals conduct electricity. Also, if the electrons are given extra
energy by heating one end of a metal bar they are free to carry to
energy down the bar, this is what makes metals good conductors of
heat.
If enough energy is given to a particular electron it can escape
the metal altogether and move off into free space. This energy can be
given to the electrons in a number of ways and one of which is by the
absorption of energy carried by light which is shone on the metals
surface.
As the metal plate is illuminated electrons can absorb enough energy
to leave the surface. They are accelerated across the potential
difference supplied by the battery of cells and current flows in the
circuit. The current is registered by the ammeter and when the light
source is removed the current stops.
Please move mouse over image.

What do experiments show about the photo-electric effect?
If light of a certain frequency (monochromatic) illuminates a metal
surface and the kinetic energy of the ejected electrons measured a
number of very important facts emerge.
(1) It does not take longer for electrons to be ejected when the
incident intensity is much reduced, there are simply less of them and
increasing the intensity of incident light increases the number of
ejected electrons but not their average kinetic energy.
(2) Below a certain frequency threshold (which is dependent on the
metal) there are no ejected electrons even with very intense
illumination.
(3) Above this frequency threshold the average
kinetic energy of the ejected electrons increases linearly with
increasing frequency.

Why are these observations so important?
It is these facts which make the photo-electric effect impossible to
explain by considering light purely as a wave phenomenon and they also
tell us about how the energy of photons is determined.
It is clear from (1) that the energy is not continuously supplied to
the electron as would be the case with wave transport but that the
electrons are receiving energy in fixed packets.
The electrons need a certain amount of energy to escape the metal,
this is known as the work function, and with this in mind point (2)
tells us that the energy they receive is in some way related to the
frequency of the incident light.
Observation (3) confirms that the energy is linearly related to
frequency.
The importance of photo-electric effect is highlighted by
the fact that it is for work in this area that A. Einstein won the
Nobel prize.

How is a photon's energy determined?
Another problem with the
wave concept of light is in explaining the radiation given out by
stars and other hot bodies. A good way to generate light is simply to
heat an object. This is how a tungsten filament light bulb works. If
we heat an object more and more we can see its colour change as it
moves from being invisible to red hot to white hot. The gas at the
centre of galaxy clusters and on the surface of stars where matter is
accreting from a companion star the temperatures can be sufficiently
hot that most of the radiation is emitted at X-ray wavelengths and XMM
will be able to study these in detail. The spectrum of radiation
depends on the temperature and the overall characteristic profile is
known a black body curve. In fact when a light bulb is switched off it
still radiates by virtue of it being at room temperature however the
radiation is at wavelengths we are unable to see. The exact spectrum
of the radiation emitted by a body because of its temperature cannot
be explained if the energy is being radiated as a wave. However,
M. Planck demonstrated how by supposing the energy was released in
fixed quantities the problem could be solved. He called these fixed
quantities of energy quanta and each quanta of energy is carried away
by a photon. The energy of a photon (E) is linearly related to the
frequency of the light (v) and the constant of proportionality is
known as Planck's constant (h) such that E=hv. Planck's constant is
around 6.626*10-34 Js.
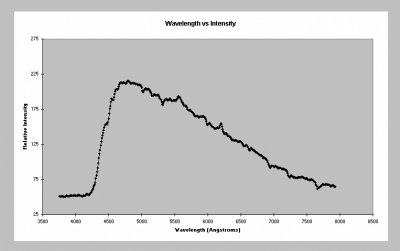
This blackbody curve was produced by analysing
the spectrum produced by the sun.
Click on picture for a higher resolution image

So, is light energy transported by a wave or a particle?
There is no single answer, it is impossible to describe all of the
observed characteristics of light with just one or other
model. Sometimes light exhibits wave like characteristics such as
interference, diffraction and refraction and in other situations its
particle like characteristics become apparent, such as in the
photo-electric effect and in describing black body radiation. Whilst
this might seem as though we do not have a coherent understanding of
light this curious dual nature of radiation is an important and
fundamental component of the physics of quantum mechanics. Light is not
transported by one or other mechanism but is simultaneously transported
by both and it is only when we perform observations or experiments that
particular characteristics become apparent. Wave-particle duality, for
particles as well as photons, is a necessary component of quantum
mechanics which is currently the most successful theory we have for
describing the behaviour of light and matter.
The End of the PHOTONS AND LIGHT topic.
|