Gas Pressure and Density

Why is Gas Pressure of importance to XMM?
XMM is an X-ray satellite and one of its main activities will be observing regions of hot gas throughout the Universe. This gas, with temperatures from 1 million to 100 million degrees Kelvin, is spread throughout the Universe, from regions close to stars, throughout the interstellar medium of our own Galaxy, and nearby galaxies, through to vast accumulations of hot gas around clusters of galaxies. The density of this gas also varies dramatically, from incredibly low densities in clusters of galaxies (a number density of 1 particle per 103 cm3, corresponding to a mass density of 10-27 gm cm-3 ) up to higher density regions around stars, with densities of 1012 particles cm-3 or even higher). Determining the density and pressure of such regions is an important part of the XMM mission, and to our understanding of the cosmos.
Gas pressure is both an everyday concept that we are confronted with on a day to day basis (such as in the weather reports, where the pressure today might be reported as 1020 millibars and so on) but also an important part of the physics of the Universe at large, and one where the XMM satellite will make a major contribution.
The XMM satellite will be observing material throughout the Universe at a phenomenal range of densities and pressures, from extremely diffuse but hot gas extending for vast regions between associations of galaxies through to very dense (and hot) gas in the vicinity of compact objects such as neutron stars.

Some Basic Concepts
The pressure exerted by a gas is, at its simplest, the simple ratio of the force exerted on a surface by the gas, divided by the area that the force is exerted over. Consequently, the units of pressure are then in units of N m-2 . The basic SI unit is the Pascal (Pa) which is equal to a pressure of 1 N m-2.

So what is Gas Pressure really?
Gas contains molecules that are in motion. The kinetic motion of these molecules (bouncing off a surface) exerts a force on that surface. From the motion of the molecules we can see that several things will affect the forces exerted by the gas.
Temperature: When a gas becomes hotter the gas particles move more rapidly. Consequently, when they collide with a surface the force they exert is greater than if the gas was cooler.
Density: Gas density is a measure of the number of particles (sometimes given as the mass density - kg m-3 or indeed gm cm-3 or as the number density m-3 - the number of particles in a given volume. If the density is higher the more particles are exerting a force then clearly the pressure is higher.
For an ideal gas (essentially just a normal gas) the equation for the gas pressure is just:
P = n kT
where T is the temperature and n is the number density.

Some other units of pressure
To complicate matters, physicists and astrophysicists often use different units for pressure. The SI unit of pressure is the Pascal = 1 N m-2.
A pressure of 1Pa is a rather small pressure, and the atmospheric gas pressure at the Earth's surface (which is often referred to as 1 atmosphere, or 1 bar) is equal to approximately 105 Pa.
In weather forecasts you will often have reference to a pressure of 1000 millibar. A millibar is equal to around 100 Pa.

Pressure in our atmosphere
The gas pressure (and density) in our own atmosphere is not constant, but falls off with height (and can be noticeably different at the top of mountains). The distribution of gas pressure is often referred to an exponential atmosphere, with a scale height (usually referred to as H) of around 8 km. This means that the gas pressure is given by the following equation:
P(h)= P(0) exp(-h/H)
where P(h) is the pressure at a height h in the atmosphere, and P(0) is the pressure at ground level.
The distribution of pressure is a consequence of a balance between forces, with the Earth's gravity pulling the gas particles down and the gas pressure (or more precisely, the gas pressure gradient) supporting them against the force of gravity.
This competition between forces applies in many places throughout the Universe. For example in stars, or indeed out Sun, there is a similar situation. The huge gravitational force of the Sun tends to want to pull all the material into the centre. Although the pressure and density at the centre of the Sun is very high (a pressure of 1016 Pa and a mass density of 105 kg m-3, the latter being 100 times greater than the density of water, a fluid) the very high temperatures (107 K) means that the Sun is gaseous throughout. Consequently stars can be thought of as balls of gas held together by gravity, but held up from collapsing by pressure.
When the forces get out of balance, spectacular things can happen. If the nuclear reactions are no longer sufficient to maintain the pressure gradient, gravity will win out and the star will contract. On the other hand, if the pressure forces become too large then the star can swell up and eject the outer layers of the star. This sort of competition between forces can result in the formation of black holes (if gravity wins) and supernova explosions (if pressure wins).
As mentioned above the pressure in the Earths atmosphere is around 105 kPa at the surface, and with a density of around 1 kg m-3.
At the typical altitudes of orbiting satellites in low Earth orbit (say 500km) the pressure is down to 10-8 Pa, and the gas density is about 10-15 gm/cm-3. XMM operates at much higher altitudes above the Earth's surface than this (even at the lowest point of its elliptical orbit), so that the gas pressures and densities are basically totally negligible. This is important because our atmosphere is a very efficient absorber of X-rays, and consequently we need to places X-ray telescopes above it in order to see the Universe.
The low pressure in orbit also has other important consequences for the XMM satellite itself. As soon as the satellite enters orbit, it begins a process called de-gassing, whereby residual atmosphere inside the satellite from before the launch begins to leak out into space. Gas tends to flow from higher pressure regions (inside the satellite when launched) into low pressure regions (space). This process can result in debris (dust etc) being deposited on sensitive surfaces (such as mirrors and detectors), and so care is taken to protect these surfaces with a system of doors.

A simple relationship for gas pressure
A very common example of the behaviour of gas pressure is referred to as Boyles law, named after the 17th Century scientist who discovered the relationship. Boyles law gives the relationship between pressure and volume of a gas if the temperature and amount of material are held constant.
If the volume of a container is increased, the pressure decreases.
If the volume of a container is decreased, the pressure increases.
The explanation is straightforward: Suppose the volume of the gas is increased, then this means that the gas molecules will impact the container walls less often per unit time (the density is decreased). This means the gas pressure will be less because there are less molecule impacts per unit time.
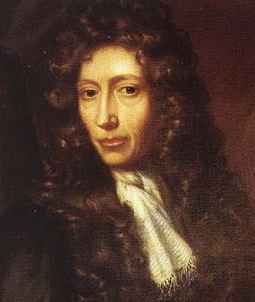
Please move mouse over image.
If the volume is decreased, the density will be increased and gas molecules have a shorter distance to go, striking the walls more often per unit time. This results in pressure being increased because there are more molecule impacts per unit time.
Boyles Law can be expressed mathematically as:
PV = constant

Pressure put to use on XMM
The CCDs and the filters in the EPIC cameras on XMM had to be protected on the ground, during launch and for the early orbits in space, principally against contamination. This was achieved by enclosing them in a housing which could be sealed and evacuated to a low pressure (~ 1 mbar). Clearly once in orbit the housing has to be opened up in some way to the cosmos in order to allow the photons reflected from the mirrors to reach the detectors. This has been done by means of a pressurised bellows door.
The unexpanded bellows is placed over the aperture and gas put into the bellows to expand it until it is jammed between the reaction pillars and the bulkhead containing the aperture to the X-ray beam. This closes off the door enabling the housing surrounding the CCD to be evacuated. In orbit the gas in the bellows is let out and the door is then free to open.
The door is pressurised on the ground to about 5 bar (5 atmospheres) differential. That is to say, the absolute pressure inside the bellows is 6 bar acting outwards; there is an outside pressure of 1 bar acting inwards giving the net differential pressure of 5 bar acting outwards. Hence when the door is placed in an evacuated chamber or in space where there is no outside atmosphere, the clamping pressure on the aperture increases to 6 bar.
Any system such as this with gas connections leaks to a greater or lesser extent. For XMM the leak specification was required to be less than 1 bar per month so that there was no chance that the door would open in an unplanned way. This is a small leak rate. Because the pressure depends on temperature, temperatures had to be monitored to a tenth of a degree so that a small drop in ambient temperature was not confused with a small leak.
But when the door is being pressurised work is being done on the gas and hence the temperature of the gas rises. Before we started to take pressure measurements therefore we had to wait about 30 minutes for the temperature of the gas to fall back to the ambient temperature (gases are not good conductors of heat).
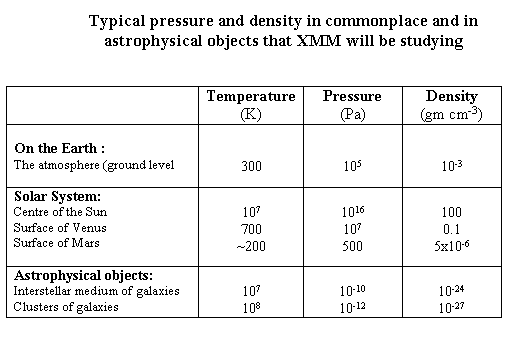
The End of the GAS PRESSURE and DENSITY topic.
|